In thermal equilibrium, the spin temperature is equal to the "real", kinetic temperature. Just after decoupling of the radiation from matter at a redshift of $z\simeq1100$, the gas and the photons share the same energy, and since $T\gg 1$, we have that $n_1/n_0 \simeq 3$. But when the first stars begin to shine, they produce massive amounts of hard UV radiation which ionizes their surrounding medium. The ionized gas quickly recombines (in the beginning, at least), with $\sim2/3$ of the recombinationrecombinations resulting in the emission of a Lyman $\alpha$ photon, i.e. a photon with an energy corresponding to the energy difference between the first excited state (one of the three $2P$ states) and the ground state (the $1S$ state) of the hydrogen atom (10.2 eV).
The Ly$\alpha$ photons scatter multiple times on the neutral hydrogen. Each scattering excites an atom from $1S\rightarrow 2P$, which subsequently de-excites and emits an Ly$\alpha$ photon in another direction. But since the energy difference between the $2P$ and the $1S$ state is a million times larger than between the hyperfine states, there is equal chance of ending in the $\uparrow\uparrow$ and the $\uparrow\downarrow$ state.
That is, $n_1/n_0$ is no longer $\simeq 3$, but is driven toward $\sim 1$. This is the Wouthuysen–Field effect that Guiseppe Rossi mentions; from eq. \ref{a}, you see that this corresponds to a much smaller spin temperature, and thus the factor that Guiseppe Rossi mentions becomes negative. The full equation describing the brightness (or, equivalently, the flux received) as a function of redshift can be written (e.g. Zaldarriaga et al. 2004)
$$
T(z) = 23\,\mathrm{mK} \,
\frac{T_S - T_\mathrm{CMB}}{T_S} \,
(1+\delta)
x_\mathrm{HI}(z)
\frac{\Omega_\mathrm{b}h^2}{0.02}
\left( \frac{0.15}{\Omega_\mathrm{m}h^2} \, \frac{1+z}{10} \right)^{1/2}\label{b}\tag{2}
$$
and when the $\frac{T_S - T_\mathrm{CMB}}{T_S}$$(T_S - T_\mathrm{CMB})\,/\,T_S$ factor is negative, you will get an absorption line.
Now the big question is, according to eq. \ref{b} the dip should be of the order a few tens of mK, but is in fact roughly 0.5 K, i.e. an order of magnitude larger. One possible mechanism that could produce this effect is coupling of the gas with dark matter, something which is not usually considered possible but could happen if the dark matter particle has a very small charge (Barkana et al. 2018).
Time evolution of the 21 cm signal
The figure below (from a great review by Pritchard & Loeb 2012) shows how the 21 cm signal evolves with time. The dip starts at "First galaxies form" and begins to disappear when "Heating begins".
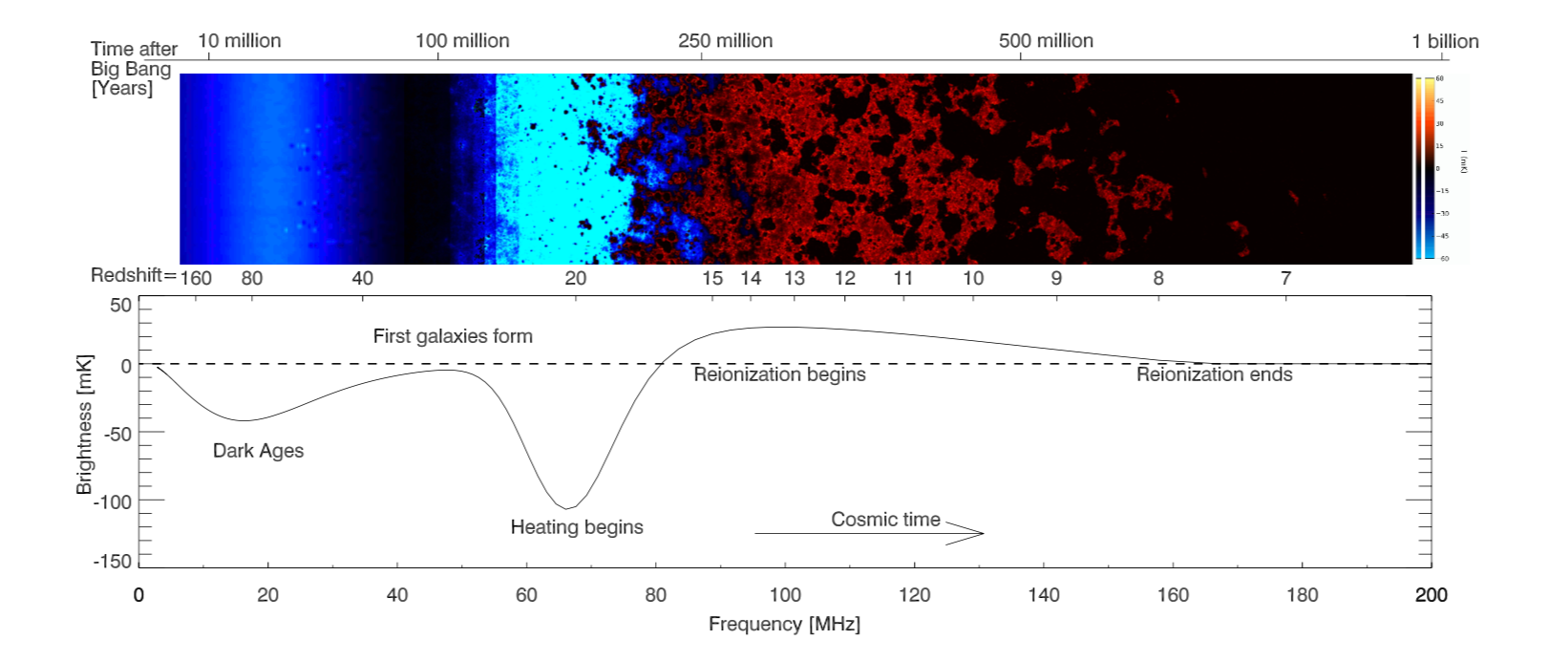